Magnetic shape memory
Magnetic shape memory (MSM) alloys exhibit a remarkable functional property to generate macroscopic strains upon exposure to a magnetic field of sufficient strength. The strong coupling between the structural and magnetic degrees of freedom gives rise to the magnetically induced reorientation (MIR) of the twinned martensitic microstructure, resulting in magnetic field-induced strain (MFIS). Strain values above 10% were reported for Ni-Mn-Ga-based single crystals, boosting the research interest in MSM materials, which have a strong application potential in sensing and actuation systems.
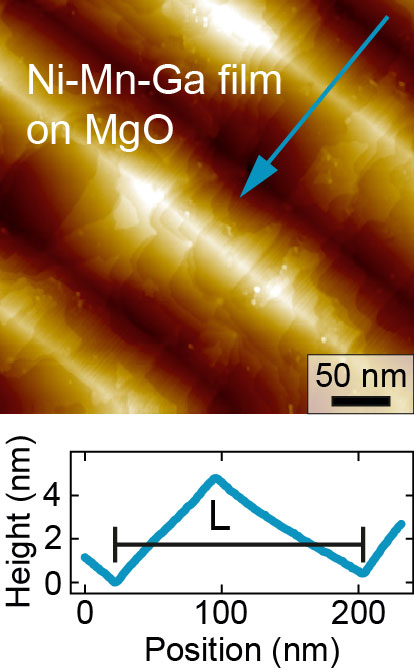
Unlike the magnetostrictive phenomenon generally occurring in structurally homogeneous samples, the MSM effect requires a special microstructure, i.e. twinning. It is formed by a displacive, diffusion-free structural transformation from a higher symmetry cubic austenite phase to a lower symmetry orthorhombic or tetragonal martensite phase. The twinning emerges in the martensite phase to minimize the total shape change and thus the macroscopic strain energy over the whole sample. The twin boundary remains the lattice of the parent phase and separates two structural domains, namely twin variants with different, but symmetrical, lattice orientations (see Figure on the right). Due to the high mobility of the twin boundaries, a sample in the martensite phase can easily be deformed. Additionally, it is necessary for MSM alloys that the magnetic anisotropy energy is higher than the energy needed to move the twin boundaries, so that the twin variants are redistributed or reoriented in applied magnetic fields and then aligned in the magnetic easy axis. In the case of nanotwins caused by layer modulations, the movement of the twin boundaries becomes easier and thus can be driven by moderate magnetic fields.
Our research is focused on the investigation of structural, electronic and magnetic properties of Ni-Mn-Ga-based MSM materials. We implement temperature dependent scanning tunneling microscopy in order to investigate the surface structure of Ni-Mn-Ga alloys in both austenitic and martensitic phases giving a possibility to address the surface structure from the micrometer scale down to the atomic level. The electronic properties of the Ni-Mn-Ga-based compounds are studied by means of angle-integrated and angle-resolved photoemission spectroscopy. We also address the fabrication and investigation of freestanding Ni-Mn-Ga thin film microstructures (see Figure below), relevant for device applications.
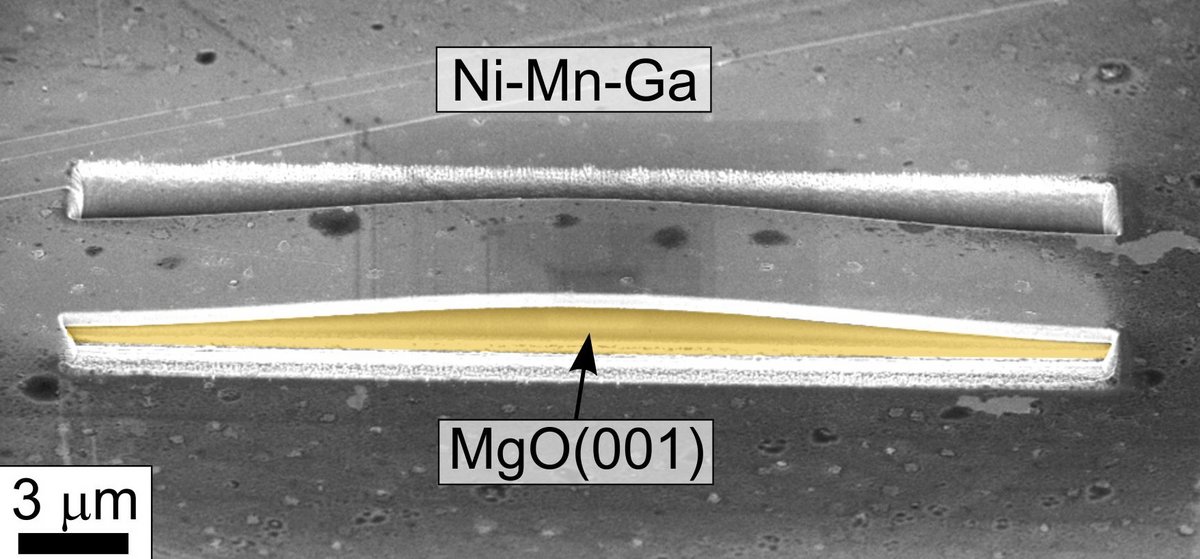